In simple terms, a LIDT (laser-induced damage threshold) reference serves as a basis of estimating how powerful a laser has to be before it begins to cause damage in an optic. Understanding and optimising LIDT is paramount therefore for designing reliable laser systems, particularly in high-power applications. This paper delves into the complexities of LIDT, exploring established testing methodologies, factors influencing its value, and strategies for developing laser-resistant optical coatings.
LIDT, or laser-induced damage threshold, is defined as the “highest quantity of laser radiation incident upon the optical component for which the extrapolated probability of damage is zero” by ISO 21254, or in other words it. LIDT is typically defined in units of fluence, J/cm^2.
ISO 21254 describes the methodology, principles and terminology of laser damage tests. Laser damage tests are typically performed in the format of 1:1 or S:1 tests. 1:1 tests consist of exposing a previously unexposed site to a single laser shot with a specified fluence. S:1 tests consist exposing a previously unexposed site to multiple consecutive laser shots with a specified fluence. ISO specifies that at least 10 sites are tested in order to gain a better representation of the LIDT.
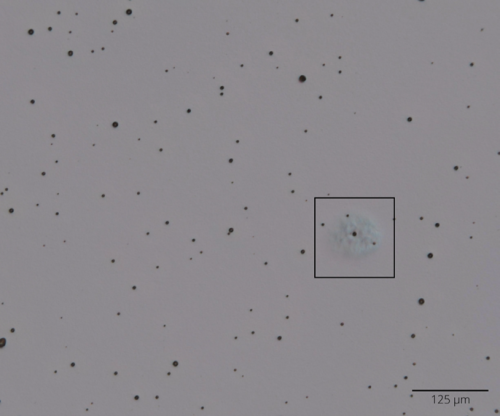
The LIDT is estimated by increasing the fluence and observing resultant changes in the coatings. In some cases any observable change in the coatings is considered a failure. An example is given in figure 1.
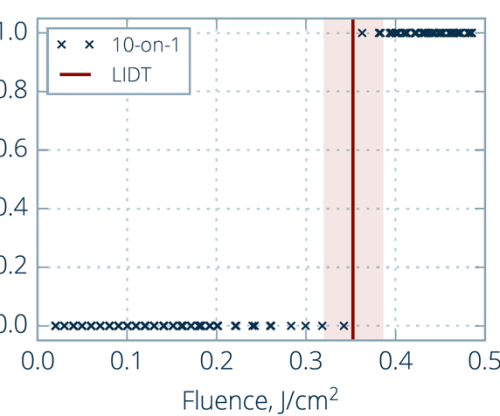
Estimates of LIDT may be influenced by a number of factors such as the cleanliness of the testing facility as well as effects such as laser conditioning. The pulse diameter and duration are also significant factors, and so LIDTs are often given with specified pulse characteristics. There are approximate methods that can be used to scale LIDT for lasers with different specifications. Within the nanosecond/microsecond regime one can estimate LIDT for a certain wavelength, diameter and pulse duration from an LIDT given with a different set of parameters. The scaling law is given by the equation below.

Sub-nanosecond scale pulses do not obey this scaling law as different damage mechanisms dominate for such short pulse durations. While LIDT in the femtosecond regime appears to be strongly related to the electron band gap of the materials, thereby providing a ‘natural’ upper limit to the LIDT, the LIDT in the pico-second regime is still far from being fully understood. As such, the exact nature of laser damage is still being actively researched. The main culprits are defects/contamination within the optical coating, coating material absorption and the resulting response of the coating to high electric field densities. For example, absorbing defects can be heated to a plasma state causing a circular pit to form as the top-most layers fail.
The exact nature of laser damage is still being actively researched. The main culprits are defects/contamination within the optical coating and the response of the coating to high electric field densities. For example, absorbing defects can be heated to a plasma state causing a circular pit to form as the top-most layers fail. Nodule defects formed during the coating progress can be ejected by the pulse leaving pits that penetrate deeply into the coating. These mechanisms can be mitigated in a multitude of ways, a common method is the addition of a silica overcoat. Another technique is to modify layer thickness’ in such a way that peaks of standing wave electric fields are reduced in high index materials as these standing waves are responsible for a number of damage mechanisms. Additionally, one can aim to reduce contamination and defects by maintaining a clean coating environment and making use of energetic coating methods.
At MPO we are constantly experimenting with laser resistant coatings and work with LIDT testing companies to achieve the highest thresholds possible.
References and Further reading
Lasers and Laser Related Equipment – Test Methods for Laser-Induced Damage Threshold ISO 21254-2:2011 (E) (2011)
Optical Interference Coatings, N. Kaiser, H. K. Pulker, Springer-Verlag Berlin Heidelberg 2003, ISBN 3-540-00364-9
Laser-Induced Damage of Optical Coatings, R. Siew, C. Hanson, T. Erdogan, 200 Dorado Place SE, Albuquerque, New Mexico 87123, CVI Laser Optics 2013.
Optical Coating Design with Reduced Electric Field Intensity, J. H. Apfel, Appl. Opt. 16: 1880-1885 (1977)
Role of Cracks, Pores and Absorbing Inclusions on Laser-Induced Damage Thresholds at Surfaces of Transparent Dielectrics, N. Bloembergen, Appl. Opt.12:661-664 (1973)
Laser-Induced Damage of Optical Elements – A status report, A. J. Glass, A. H. Guenther, Appl. Optics 12: 637 (1973)
Laser Damage in Optical Materials, R. M. Wood, Adam Hilger Series on Optics and Optoelectronics (1986)